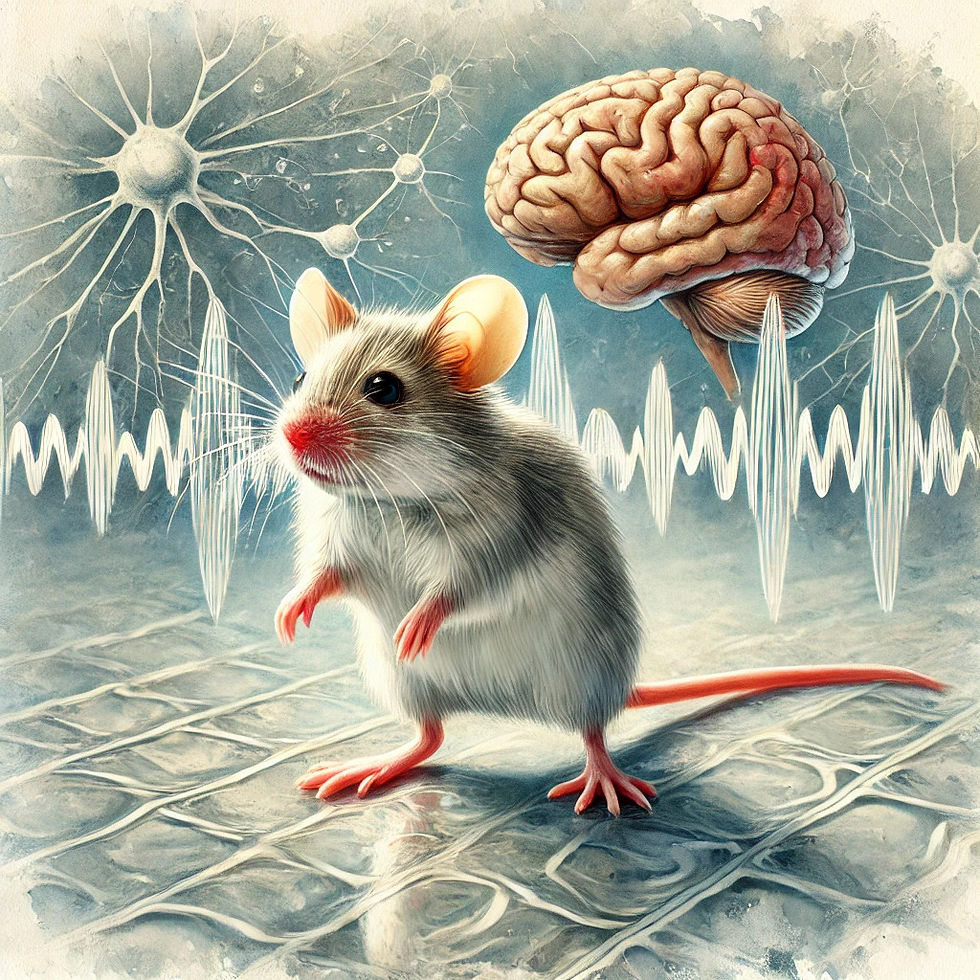
Here is an introduction for the general readers. We hope to make science more accessible!
As someone approaches, a little mouse senses changes in its surroundings. Alert and on edge, it must decide whether to flee. But how exactly does the mouse detect the environmental changes? Beyond the obvious mechanisms like visual detection of light or auditory perception of sound, there’s another crucial sense at play: somatosensory system detecting vibrations through the ground.
The mouse's hind feet, resting against the floor, house an array of extraordinarily sensitive end-organs, from the toes to the leg bones. These specialized structures can detect minuscule vibrations as small as fractions of a micron. The end-organs convert vibrations into neural electrical signals, which are then transmitted to the central nervous system.
Vibrations traveling through the ground might signal a potential threat or a call from a fellow mouse. This "substrate-borne vibration" is a critical topic in tactile research, as it sheds light on how animals perceive their environments and ensure survival. While the environment can be endlessly complex, a vibration signal can be described using a few fundamental physical quantities: the amplitude, the frequency, and the location where the input occurs. How does a mouse's nervous system encode the position and frequency of vibrations, and how are these two types of information integrated? Let’s break it down.
Mapping the Position: Somatotopy in the Nervous System
The position of a vibrational stimulation is encoded in the nervous system through "somatotopy," a mapping of the body’s surface onto neural systems. The most familiar example is the homunculus (Latin for "little man") in the brain’s primary sensory cortex. This iconic illustration depicts different parts of the human body exaggerated in proportion to their sensory importance. Though distorted, adjacent body parts on the homunculus correspond to adjacent regions in the brain—still resembling a recognizable figure.
But this type of organization isn’t limited to the cerebral cortex. Our recent study has found similar somatotopic mapping in the brainstem of mice. Applying vibrations to different spots along a mouse’s leg—from toes to thighs—we observed that corresponding regions in the brainstem were activated in a systematic way.
As tactile signals travel from peripheral nerves to the brain, they pass through the spinal cord, brainstem, thalamus, and finally the sensory cortex. Interestingly, somatotopy is almost undetectable at the spinal cord in mice, but it suddenly becomes prominent in the brainstem before persisting—albeit slightly weakened—through the thalamus and cortex.
This consistent spatial organization reflects a common strategy of the nervous system: encoding continuous physical quantities in a structured way. Not just space—frequencies of vibration are also mapped systematically, akin to a frequency "map."
Mapping Frequency: The Role of Tonotopy
Just as somatotopy organizes spatial input, tonotopy organizes frequency. The best-known example is the cochlea, the spiral-shaped organ in the inner ear. Neurons in the cochlea are arranged in a gradient: high-frequency-sensitive cells are located at the base, while low-frequency-sensitive ones cluster near the apex. This layout essentially forms a "frequency map."
While tonotopy is crucial for hearing, it also plays a key role in touch. Vibrations detected by a mouse’s hind limbs are encoded into a "frequency map" that appears in the brainstem and is preserved through the thalamus and sensory cortex. Scientists speculate that tactile tonotopy complements auditory perception, extending a mouse’s sensory range. For instance, mice hear sound waves in the 3,000–60,000 Hz range, while their tactile sensors cover a lower frequency range of 100–3,000 Hz. Together, these systems form a seamless sensory partnership.
Overlapping Maps in the Brainstem
Here’s where it gets fascinating: both somatotopy and tonotopy exist simultaneously in the mouse’s brainstem—and the two maps overlap almost perfectly. In the dorsal column nuclei (DCN) of the brainstem, neurons at the anterior end respond to vibrations near the toes and of low-frequency, while neurons at the posterior end react to vibrations at the thigh and of high-frequency. This striking overlap suggests a functional link between spatial and frequency information.
One reason for this overlap could lie in the highly specialized tactile receptors in the mouse’s hind legs. Each less than a millimeter in size, these tiny sensory organs, known as low-threshold mechanoreceptors (LTMRs), are tuned to specific frequency ranges. For example, Pacinian corpuscles are highly sensitive to high frequencies (around 1,000 Hz for mouse), while Merkel cells specialize in detecting low frequencies, and Meissner corpuscles respond to medium-to-low frequencies. These receptors are distributed strategically: Merkel cells are concentrated near the toes, while Pacinian corpuscles are found in the periosteum of the leg bones—mirroring the brainstem’s maps of low and high frequencies, respectively.
However, there’s a twist: these maps don’t appear in the spinal cord (the first stop for sensory signals). They only emerge in the brainstem. This means that while LTMRs distribution helps explain the patterns, it cannot fully account for the emergence and the overlap between somatotopy and tonotopy. Neural connections within the brainstem itself likely play a crucial role—a topic ripe for further investigation.
Beyond a Relay: The Brainstem as a Sensory Processor
This discovery challenges the traditional view of the brainstem as a mere relay station for sensory signals. Instead, it highlights the brainstem as a key site for integrating tactile information, enabling mice to interpret their environment with remarkable precision. Vibrations transmitted through the ground might signify the presence of a predator, a precariously swaying branch, or the texture of the terrain underfoot.
Tactile signals, like sound and light, are composed of waves and can be described using simple parameters like amplitude, location, and frequency. Just as the eyes are the windows to the soul and the ears the pathways to connection, the body's tactile receptors are like an infrared detection system that allows animals to perceive their surroundings in 360 degrees of clarity. It is this intricate tactile network that gives creatures a profound sense of presence in the world.
Comments